Projects
Project 1: Regulation of skeletal development
The integration of mechanical forces and genetic regulation of skeletal development.
This research aims to discover the molecular mechanisms involved in regulating the correct formation of the skeleton in the embryo. In particular we focus on investigating how developing skeletal tissues respond to mechanical stimuli generated by embryonic muscle contractions during development. We know that the movement of the human embryo in the uterus (experienced as the baby “kicking” in the womb), or chick embryos inside the incubated egg, is needed for the formation of normal bones and joints. In infants that experience reduced movement for a variety of reasons, bones are thin and weak. We have been investigating the basis of this observation in mouse and chick embryos. Using an interdisciplinary approach together with Bioengineers, our work showed that mechanical stimulation from contracting muscles in the early embryo is important for normal bone formation with delayed ossification and failure of joint formation in the absence of contractions (Figure 1 and(refs 1,2,3 below). We used novel computational modelling to predict how mechanical forces impact developing tissues (refs 4,5,6) and we showed that specific developmental regulatory genes are affected when the mechanical environment is altered (refs 1,3,4). We propose that the cells of the skeletal tissues require mechanical stimulation to differentiate appropriately. We are now examining the response of embryonic cells in the embryo to mechanical stimulation at a molecular level, revealing the genes that are switched on and off in response to mechanical stimulation. This work asks questions about the control of cartilage and bone differentiation in the embryo and works toward the application of this knowledge to regenerative therapies. In the field of tissue engineering there is widespread interest in incorporating mechanical stimuli into regimes for regenerating skeletal tissues from progenitor cells, however we need to know more about how mechanical stimuli operate in normal development.
Figure 1: Figure 1: Lack of mechanical stimulation from muscle contractions impacts several aspects of skeletal development: the mouse forelimb and chick hindlimb are used here to summarise the effects on (a) ossification, (b) joint formation and (c) rudiment shape (Morphogenesis). Rolfe et al. (2013)Curr Osteoporos Rep. 11:107-16
This work has been carried out in collaboration with Patrick Prendergast and Daniel Kelly at the Trinity Centre for BioEngineering (TCBE).
VIDEO CLIP: See this small video clip by Rebecca Rolfe about her work as part of the ongoing project at http://www.youtube.com/watch?v=S-8nn7mRInU

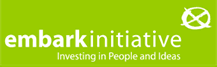

The work was funded by a The Wellcome Trust, The Irish Research Council and Trinity College Studentships
Our key publications for project 1
- Rolfe et al., Identification of mechanosensitive genes during skeletal development: alteration of genes associated with cytoskeletal rearrangement and cell signalling pathways BMC Genomics 2014
- Roddy, K.A., Prendergast P. And Murphy P. (2011) PLoS One. 2011 Feb 28;6(2):e17526
- Nowlan, N. C., Bourdon C, Tajbakhsh S, Dumas G, Prendergast PJ, and Paula Murphy, (2010) Bone 46(5):1275-85.
- Nowlan, N., Prendergast P.J. and Murphy, P. (2008) PLOS Computational Biology 4(12):e1000250.doi.1371/journal.pcbi.1000250.
- Nowlan NC, Dumas G, Tajbakhsh S, Prendergast PJ, and Paula Murphy (2012) Biomechanics and Modeling in Mechanobiology (BMMB) 207-219
- Roddy K.A., Kelly, G.M., van Es M.H., Murphy, P. and Prendergast P. (2011) Journal of Biomechanics 44(1):143-9.
- Nowlan N., Murphy P., and Prendergast P. (2008). Journal of Biomechanics 41(2):249-58. Nov 13th EPub doi:10.1016/j.jbiomech.2007.09.031.
Project 2: Mouse models of human congenital malformations of the digestive and respiratory tracts.
One in about 3500 newborn infants suffer from abnormalities called Oesophageal Atresia (OA) and/or Trachea-Oesophageal Fistula (TOF), where the oesophagus does not connect with the stomach (OA) and/or there is an abnormal connection between the oesophagus and the respiratory tract. This means that about 20 infants are born with these conditions in Ireland every year. Surgical intervention can successfully repair the gap, but there are often health issues in later life. The aim of this work is to uncover the early anomolies during embryogenesis that can lead to such abnormalities of the digestive and respiratory tracts.
![]() |
Figure 2: 3D imaging of the developing digestive and respiratory tracts as well as the floor plate and notochord using expression of Hnf3b and Optical Projection Tomography (OPT) imaging. The three images are external views of the same 3D computer representation taken form different perspectives. st, stomach; fp and nc, floorplate and notochord; lb, lung buds; hg, hindgut. see Hajduk et al., (2011) PLoS ONE 6: e27635
|
Using a mouse model and a combination of molecular techniques and 3D imaging (Figure 2) we have shown that a common early abnormality (98% of embryos) occurs in the notochord, an important organising tissue (Figure 3, Ref 1 below), which is branched and located abnormally close to the developing foregut, adjacent to where foregut patterning abnormalities are seen. We have further shown that normal movement of the notochord away from the developing foregut fails to occur in this model and that the characteristics of the constituent cells of the notochord are altered (Figure 4, Ref 2 below). We are now investigating the signalling pathways that are altered in the developing foregut.
The work, in collaboration with Prof Prem Puri at the National Children’s Research Centre (NCRC) in Crumlin Hospital, is funded by the NCRC
![]() |
Figure 3: Abnormal positioned notochord branches (red asterisk) in three adriamycin treated embryos, adjacent to abnormal foregut showing atresia (centre image) and stenosis (right image). fp, floorplate; nc, notochord;
|
Figure 4: Embryos exposed to adriamycin show abnormal expression of E-cadherin around notochord cells (compare a with e) and abnormal delamination away from the foregut (compare d with h) during early patterning of the foregut. nc, notochord; fg, foregut. see Hajduk et al (2012) Birth Defects Research Part B 95: 175-183
Our key publications for project 2
1. Danielle Mc Laughlin, Paula Murphy, Prem Puri Altered Tbx1 gene expression is associated with abnormal oesophageal development in the adriamycin mouse model of oesophageal atresia/tracheo-oesophageal fistula Pediatric surgery international 2014.
2. Danielle Mc Laughlin, Paula Murphy, Prem Puri Notochord manipulation does not impact oesophageal and tracheal formation from isolated foregut in 3D explant culture Pediatric surgery international 2016,
3. Hajduk P., Sato H., Puri P. and Murphy P (2011). Abnormal Notochord Branching is Associated with Foregut Abnormalities in an Adriamycin Treated Mouse Model. PLoS ONE 6: e27635:
4. Hajduk, Piotr; May, Alison; Puri, Prem; Murphy, Paula (2012) "The Effect of Adriamycin Exposure on the Notochord of Mouse Embryos" in press Birth Defects Research Part B 95: 175-183
Project 3: Relating multiple gene expression patterns to morphological changes in the developing embryo, including cross species comparisons.
The scope of questions we need to ask about development has changed. We currently know quite a bit about individual genes that are required for correct development; the challenge now is to integrate large amounts of information to extract governing principles. This was the perspective of an SFI funded project, which gathered whole embryo 3D expression data on 40 genes involved in an important cell communication system (Wnt signalling), producing 3D computer representations of the expression patterns in the context of the developing embryo (Figure 5). This work revealed a complexity in gene expression patterns not previously envisaged (ref 1,2,3 below). These data have been made fully available to the research community through the Edinburgh Mouse Atlas of Gene Expression (EMAGE), and through a local database on this website. This work led to involvement in a BBSRC funded network grant to develop the Chick Atlas of gene expression. This has allowed us to carry out cross species comparisons to examine how changes in the deployment of highly conserved regulatory genes might relate to morphological evolution (ref 4, Figure 6).
![]() |
Figure 5: A and B show developing embryos at a stage when the shape of the limbs is being determined (11.5 dpc). They reveal (dark staining) where the genes for different Wnt signalling molecules are switched on (expressed). A; Wnt11. B; Wnt6. C-F show single views of 3D computer models of the forelimb. D represents the expression of Wnt11, E of Wnt6 and F shows the merger of both. Yellow indicates overlap in the 3D expression patterns.
|
![]() |
![]() |
Figure 6: expression of the Sfrp1 gene in a chick embryo at stage HH26- 3D image 3-D |
expression of Sfrp1 in a mouse embryo at stage TS19- 3D image - such data can be sued to compare the activity of developmental regulatory genes across species |
Developing computational tools to integrate 3D data in the context of a developing embryo was a major challenge that has recently been achieved by our collaborators (EMAP) and our Wnt pathway gene data is now being mapped in 3D (see http://www.emouseatlas.org/emage/analysis/3d_mapping.html) (Figure 7) showing subdivision of the embryo into unique spatial territories based on combinations of Wnt pathway genes. This, as yet unpublished work is carried out in collaboration with Duncan Davidson, Richard Baldock, Bill Hill, Chris Armit and Venkat at the Edinburgh Mouse Atlas Project
![]() |
Figure 7: 3D mapping of multiple Wnt gene expression patterns at embryonic stage TS17 (embryonic day 10). Each colour represents the pattern of a different Wnt encoding gene. Mapping developed and achieved at the Edinburgh Mouse Atlas Project. A and B show developing embryos at a stage when the shape of the limbs is being determined (11.5 dpc).
|
Our key publications for project 3
- Summerhurst K., Stark M., Sharpe J., Davidson D., Murphy P. (2008). Gene Expression Patterns 8(5):331-48
- Fisher ME et al. (2008). Developmental Biology 317(1):13-23
- Vendrell V., Summerhurst K, Sharpe J, Davidson D, Murphy P. (2009) Gene Expression Patterns Jun;9(5):296-305
- Martin, Audrey; Maher, Stephanie; Summerhurst, Kristen; Davidson, Duncan; Murphy, Paula (2012). Evolution and Development 14: 178-195